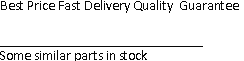
ADM1024ARU ,System Hardware Monitor with Remote Diode Thermal SensingSystem Hardware Monitor withaRemote Diode Thermal SensingADM1024
ADM1024ARUZ ,Reconfigurable Remote Temperature Sensor and Supply Voltage Monitor, Fan Control, Chassis Intrusion with Serial InterfaceSystem Hardware Monitor withRemote Diode Thermal SensingADM1024
ADM1024ARUZ ,Reconfigurable Remote Temperature Sensor and Supply Voltage Monitor, Fan Control, Chassis Intrusion with Serial InterfaceAPPLICATIONSUp to 9 Measurement Channels Network Servers and Personal ComputersInputs Programmable- ..
ADM1024ARUZ-REEL ,Reconfigurable Remote Temperature Sensor and Supply Voltage Monitor, Fan Control, Chassis Intrusion with Serial InterfaceGENERAL DESCRIPTIONOn-Chip Temperature Sensor The ADM1024 is a complete system hardware monitor for ..
ADM1025ARQ ,Low-Cost PC Hardware Monitor ASICSPECIFICATIONSA MIN MAX CC MIN MAXParameter Min Typ Max Unit Test Conditions/CommentsPOWER SUPPLYSu ..
ADM1025ARQ-REEL ,Remote Multichannel Temperature Sensor, Power Supply Voltage Monitor with Serial Interfaceapplications in personal computers,electronic test equipment, and office electronics.FUNCTIONAL BLOC ..
AH102 , Medium Power, High Linearity Amplifier
AH102A , Medium Power, High Linearity Amplifier
AH102A , Medium Power, High Linearity Amplifier
AH103A-G , High Gain, High Linearity ½-Watt Amplifier
AH103A-G , High Gain, High Linearity ½-Watt Amplifier
AH104F245001-T , CHIP ANTENNA
ADM1024ARU
System Hardware Monitor with Remote Diode Thermal Sensing
REV.0
System Hardware Monitor with
Remote Diode Thermal Sensing
FUNCTIONAL BLOCK DIAGRAM
FEATURES
Up to Nine Measurement Channels
Inputs Programmable-to-Measure Analog Voltage, Fan
Speed or External Temperature
External Temperature Measurement with Remote
Diode (Two Channels)
On-Chip Temperature Sensor
Five Digital Inputs for VID Bits
LDCM Support
System Management Bus (SMBus)
Chassis Intrusion Detect
Interrupt and Over Temperature Outputs
Programmable RESET Input Pin
Shutdown Mode to Minimize Power Consumption
Limit Comparison of all Monitored Values
PRODUCT DESCRIPTIONThe ADM1024 is a complete system hardware monitor for
microprocessor-based systems, providing measurement and limit
comparison of various system parameters. Eight measurement
inputs are provided, of which three are dedicated to monitoring
5 V and 12 V power supplies and the processor core voltage.
The ADM1024 can monitor a fourth power-supply voltage by
measuring its own VCC. One input (two pins) is dedicated to a
remote temperature-sensing diode. Two further pins can be
(continued on page 7)
APPLICATIONS
Network Servers and Personal Computers
Microprocessor-Based Office Equipment
Test Equipment and Measuring Instruments
ADM1024–SPECIFICATIONS1, 2(TA = TMIN to TMAX, VCC = VMIN to VMAX, unless otherwise noted)
ADM1024NOTESAll voltages are measured with respect to GND, unless otherwise specified.Typicals are at TA = 25°C and represent most likely parametric norm. Shutdown current typ is measured with VCC = 3.3 V.TUE (Total Unadjusted Error) includes Offset, Gain and Linearity errors of the ADC, multiplexer and on-chip input attenuators, including an external series input
protection resistor value between zero and 1 kΩ.Total monitoring cycle time is nominally m × 755 µs + n × 33244 µs, where m is the number of channels configured as analog inputs, plus two for the internal VCC
measurement and internal temperature sensor, and n is the number of channels configured as external temperature channels (D1 and D2).The total fan count is based on two pulses per revolution of the fan tachometer output.Open-drain digital outputs may have an external pull-up resistor connected to a voltage lower or higher than VCC (up to 6.5 V absolute maximum).All logic inputs except ADD are tolerant of 5 V logic levels, even if VCC is less than 5 V. ADD is a three-state input that may connected to VCC, GND, or left open-circuit.Timing specifications are tested at logic levels of VIL = 0.8 V for a falling edge and VIH = 2.2 V for a rising edge.
Specifications subject to change without notice.
Figure 1.Diagram for Serial Bus Timing
ADM1024
ABSOLUTE MAXIMUM RATINGS*Positive Supply Voltage (VCC) . . . . . . . . . . . . . . . . . . . . . 6.5 V
Voltage on 12 V VIN Pin . . . . . . . . . . . . . . . . . . . . . . . . . 20 V
Voltage on AOUT, N TEST_OUT ADD, 2.5 VIN/D2+
. . . . . . . . . . . . . . . . . . . . . . . . . . . . –0.3 V to (VCC + 0.3 V)
Voltage on Any Other Input or Output Pin . . –0.3 V to +6.5 V
Input Current at Any Pin . . . . . . . . . . . . . . . . . . . . . . . ±5 mA
Package Input Current . . . . . . . . . . . . . . . . . . . . . . . . ±20 mA
Maximum Junction Temperature (TJ max) . . . . . . . . . . 150°C
Storage Temperature Range . . . . . . . . . . . . –65°C to +150°C
Lead Temperature, Soldering
Vapor Phase 60 sec . . . . . . . . . . . . . . . . . . . . . . . . . . 215°C
Infrared 15 sec . . . . . . . . . . . . . . . . . . . . . . . . . . . . . . .200°C
ESD Rating All Pins . . . . . . . . . . . . . . . . . . . . . . . . . . .2000 V
*Stresses above those listed under Absolute Maximum Ratings may cause
permanent damage to the device. This is a stress rating only; functional operation
of the device at these or any other conditions above those indicated in the
operational section of this specification is not implied. Exposure to absolute
maximum rating conditions for extended periods may affect device reliability.
THERMAL CHARACTERISTICS24-Lead Small Outline Package: θJA = 50°C/W, θJC = 10°C/W.
ORDERING GUIDE
PIN CONFIGURATION
PIN FUNCTION DESCRIPTIONS
LEAKAGE RESISTANCE – M�
TEMPERATURE ERROR
�C30
–20Figure 2.Temperature Error vs. PC Board Track Resistance
Figure 3.Temperature Error vs. Power Supply Noise
Frequency
FREQUENCY – Hz50M500
TEMPERATURE ERROR
�C50k500k5MFigure 4.Temperature Error vs. Common-Mode Noise
Figure 5.Pentium® III Temperature Measurement vs.
ADM1024 Reading
Figure 6.Temperature Error vs. Capacitance Between D+
and D–
FREQUENCY – Hz5050M500
TEMPERATURE ERROR
�C50k500k5M
100k25MFigure 7.Temperature Error vs. Differential-Mode Noise
ADM1024–Typical Performance Characteristics
(continued from page 1)
configured as inputs to monitor a 2.5 V supply and a second
processor core voltage, or as a second temperature sensing input.
The remaining two inputs can be programmed as general-purpose
analog inputs or as digital fan-speed measuring inputs.
Measured values can be read out via an SMBus serial System
Management Bus, and values for limit comparisons can be
programmed in over the same serial bus. The high-speed
successive-approximation ADC allows frequent sampling of
all analog channels to ensure a fast interrupt response to any
out-of-limit measurement.
The ADM1024’s 2.8 V to 5.5 V supply voltage range, low supply
current, and SMBus interface make it ideal for a wide range
of applications. These include hardware monitoring and protec-
tion applications in personal computers, electronic test equipment,
and office electronics.
GENERAL DESCRIPTIONThe ADM1024 is a complete system hardware monitor for
microprocessor-based systems. The device communicates with
the system via a serial System Management Bus. The serial bus
controller has a hardwired address line for device selection (Pin
1), a serial data line for reading and writing addresses and data
(Pin 3), and an input line for the serial clock (Pin 4). All control
and programming functions of the ADM1024 are performed over
the serial bus.
MEASUREMENT INPUTSProgrammability of the measurement inputs makes the ADM1024
extremely flexible and versatile. The device has a 10-bit A-to-D
converter, and nine measurement input pins that can be configured
in different ways.
Pins 5 and 6 can be programmed as general-purpose analog
inputs with a range of 0 V to 2.5 V, or as digital inputs to
monitor the speed of fans with digital tachometer outputs. The
fan inputs can be programmed to accommodate fans with dif-
ferent speeds and different numbers of pulses per revolution from
their tach outputs.
Pins 15, 16, and 19 are dedicated analog inputs with on-chip
attenuators, configured to monitor 12 V, 5 V and the processor
core voltage, respectively.
Pins 17 and 18 may be configured as analog inputs with on-chip
attenuators to monitor a second processor core voltage and a
2.5 V supply, or they may be configured as a temperature input
and connected to a second temperature-sensing diode.
The ADC also accepts input from an on-chip bandgap tempera-
ture sensor that monitors system-ambient temperature.
Finally, the ADM1024 monitors the supply from which it is
powered, so there is no need for a separate 3.3 V analog input,
if the chip VCC is 3.3 V. The range of this VCC measurement
can be configured for either a 3.3 V or 5 V VCC by Bit 3 of the
Channel Mode Register.
SEQUENTIAL MEASUREMENTWhen the ADM1024 monitoring sequence is started, it cycles
sequentially through the measurement of analog inputs and the
temperature sensor, while at the same time the fan speed inputs
are independently monitored. Measured values from these inputs
are stored in Value Registers. These can be read out over the
serial bus, or can be compared with programmed limits stored in
the Limit Registers. The results of out-of-limit comparisons are
stored in the Interrupt Status Registers, and will generate an
interrupt on the INT line (Pin 10).
Any or all of the Interrupt Status Bits can be masked by appro-
priate programming of the Interrupt Mask Register.
PROCESSOR VOLTAGE IDFive digital inputs (VID4 to VID0—Pins 20 to 24) read the
processor voltage ID code. These inputs can also be reconfigured
as interrupt inputs.
The VID pins have internal 100 k� pull-up resistors.
CHASSIS INTRUSIONA chassis intrusion input (Pin7) is provided to detect unauthorized
tampering with the equipment.
RESETA RESET input/output (Pin 12) is provided. Pulling this pin low
will reset all ADM1024 internal registers to default values. The
ADM1024 can also be programmed to give a low-going 45 ms
reset pulse at this pin.
The RESET pin has an internal, 100 kΩ pull-up resistor.
ANALOG OUTPUTThe ADM1024 contains an on-chip, 8-bit digital-to-analog
converter with an output range of zero to 2.5 V (Pin 11). This is
typically used to implement a temperature-controlled fan by
controlling the speed of a fan dependent upon the temperature
measured by the on-chip temperature sensor.
Testing of board level connectivity is simplified by providing a
NAND tree test function. The AOUT (Pin 11) also doubles as
a NAND test input, while Pin 1 doubles as a NAND tree output.
INTERNAL REGISTERS OF THE ADM1024Figure 8.Standby Current vs. Temperature
ADM1024
Configuration Registers:Provide control and configuration.
Channel Mode Register:Stores the data for the operating
modes of the input channels.
Address Pointer Register:This register contains the address that
selects one of the other internal registers. When writing to the
ADM1024, the first byte of data is always a register address, which
is written to the Address Pointer Register.
Interrupt (INT) Status Registers:Two registers to provide
status of each Interrupt event. These registers are also mirrored
at addresses 4Ch and 4Dh.
Interrupt (INT) Mask Registers:Allow masking of individual
interrupt sources.
Temperature Configuration Register:The configuration of
the temperature interrupt is controlled by the lower three bits of
this register.
VID/Fan Divisor Register:The status of the VID0 to VID4
pins of the processor can be written to and read from these reg-
isters. Divisor values for fan-speed measurement are also stored
in this register.
Value and Limit Registers:The results of analog voltage
inputs, temperature and fan speed measurements are stored in
these registers, along with their limit values.
Analog Output Register: The code controlling the analogoutput DAC is stored in this register.
Chassis Intrusion Clear Register:A signal latched on the
chassis intrusion pin can be cleared by writing to this register.
SERIAL BUS INTERFACEControl of the ADM1024 is carried out via the serial bus. The
ADM1024 is connected to this bus as a slave device, under the
control of a master device, e.g., ICH.
The ADM1024 has a 7-bit serial bus address. When the device
is powered up, it will do so with a default serial bus address. The
five MSBs of the address are set to 01011, the two LSBs are
determined by the logical states of Pin 1 (NTESTOUT/ADD).
This is a three-state input that can be grounded, connected to
VCC or left open-circuit to give three different addresses.
Table I.ADD Pin Truth TableIf ADD is left open-circuit the default address will be 0101100. ADD
is sampled only at power-up, so any changes made while power is
on will have no immediate effect.
The facility to make hardwired changes to A1 and A0 allows the
user to avoid conflicts with other devices sharing the same serial
bus, for example if more than one ADM1024 is used in a system.
The serial bus protocol operates as follows:The master initiates data transfer by establishing a START
condition, defined as a high-to-low transition on the serial
condition, and shift in the next eight bits, consisting of a
7-bit address (MSB first) plus an R/W bit, which determines
the direction of the data transfer, i.e., whether data will be
written to or read from the slave device.
The peripheral whose address corresponds to the transmitted
address responds by pulling the data line low during the low
period before the ninth clock pulse, known as the Acknowledge
Bit. All other devices on the bus now remain idle while the
selected device waits for data to be read from or written to it.
If the R/W bit is a 0, the master will write to the slave device.
If the R/W bit is a 1, the master will read from the slave device.Data is sent over the serial bus in sequences of nine clock
pulses, eight bits of data followed by an Acknowledge Bit
from the slave device. Transitions on the data line must occur
during the low period of the clock signal and remain stable
during the high period, as a low-to-high transition when the
clock is high may be interpreted as a STOP signal. The number
of data bytes that can be transmitted over the serial bus in
a single READ or WRITE operation is limited only by what the
master and slave devices can handle.When all data bytes have been read or written, stop conditions
are established. In WRITE mode, the master will pull the
data line high during the 10th clock pulse to assert a STOP
condition. In READ mode, the master device will override
the acknowledge bit by pulling the data line high during the
low period before the ninth clock pulse. This is known as No
Acknowledge. The master will then take the data line low
during the low period before the tenth clock pulse, then high
during the tenth clock pulse to assert a STOP condition.
Any number of bytes of data may be transferred over the serial
bus in one operation, but it is not possible to mix read and write
in one operation because the type of operation is determined at
the beginning and cannot subsequently be changed without
starting a new operation.
In the case of the ADM1024, write operations contain either
one or two bytes, and read operations contain one byte and
perform the following functions.
To write data to one of the device data registers or read data
from it, the Address Pointer Register must be set so that the
correct data register is addressed, then data can be written into
that register or read from it. The first byte of a write operation
always contains an address that is stored in the Address Pointer
Register. If data is to be written to the device, the write operation
contains a second data byte that is written to the register
selected by the address pointer register. This is illustrated in
Figure 9a. The device address is sent over the bus followed by
R/W set to 0. This is followed by two data bytes. The first data
byte is the address of the internal data register to be written
to, which is stored in the Address Pointer Register. The second
data byte is the data to be written to the internal data register.
When reading data from a register there are two possibilities:If the ADM1024’s Address Pointer Register value is unknown
or not the desired value, it is first necessary to set it to the
correct value before data can be read from the desired data
register. This is done by performing a write to the ADM1024
A read operation is then performed consisting of the serial bus
address, R/W bit set to 1, followed by the data byte read from
the data register. This is shown in Figure 9c.If the Address Pointer Register is known to be already at the
desired address, data can be read from the corresponding
data register without first writing to the Address Pointer
Register, so Figure 9b can be omitted.
NOTESAlthough it is possible to read a data byte from a data register
without first writing to the Address Pointer Register, if the
Address Pointer Register is already at the correct value, it is
not possible to write data to a register without writing to the
Address Pointer Register because the first data byte of a write is
always written to the Address Pointer Register.In Figures 9a to 9c, the serial bus address is shown as the
default value 01011(A1)(A0), where A1 and A0 are set by
the three-state ADD pin.
Figure 9a.Writing a Register Address to the Address Pointer Register, then Writing Data to the Selected Register
Figure 9b.Writing to the Address Pointer Register Only
Figure 9c.Reading Data from a Previously Selected Register
Pins 13 and 14 are dedicated to temperature measurement, while
Pins 15, 16, and 19 are dedicated analog input channels. Their
function is unaffected by the Channel Mode Register.
Pins 5 and 6 can be individually programmed as analog inputs,
or as digital fan speed measurement inputs, by programming
Bits 0 and 1 of the Channel Mode Register.
Pins 17 and 18 can be configured as analog inputs or as inputs
for external temperature-sensing diodes by programming Bit 2
of the Channel Mode Register.
Bit 3 of the Channel Mode Register configures the internal VCC
measurement range for either 3.3 V or 5 V.
Bits 4 to 6 of the Channel Mode Register enable or disable Pins
22 to 24, when they are configured as interrupt inputs by setting
Bit 7 of the Channel Mode Register. This function is controlled
for Pins 20 and 21 by Bits 6 and 7 of Configuration Register 2.
Bit 7 of the Channel Mode Register allows the processor core
voltage ID bits (VID0 to VID4, Pins 24 to 20) to be reconfigured
as interrupt inputs.
ADM1024
Table II.Channel Mode RegisterPower-on Default = 0000 0000
Table III.A/D Output Code vs. VIN
A-TO-D CONVERTERThese inputs are multiplexed into the on-chip, successive
approximation, analog-to-digital converter. This has a resolution
of eight bits. The basic input range is zero to 2.5 V, which is
the input range of AIN1 and AIN2, but five of the inputs have
built-in attenuators to allow measurement of 2.5 V, 5 V, 12 V
and the processor core voltages VCCP1 and VCCP2, without any
external components. To allow for the tolerance of these supply
voltages, the A-to-D converter produces an output of 3/4 full-scale
(decimal 192) for the nominal input voltage, and so has adequate
headroom to cope with overvoltages. Table III shows the input
ranges of the analog inputs and output codes of the A-to-D
converter.
When the ADC is running, it samples and converts an input
every 748 µs, except for the external temperature (D1 and D2)
inputs. These have special input signal conditioning and are
averaged over 16 conversions to reduce noise, and a measure-
ment on one of these inputs takes nominally 9.6 ms.
INPUT CIRCUITSThe internal structure for the analog inputs are shown in Figure
10. Each input circuit consists of an input protection diode,
an attenuator, plus a capacitor to form a first-order low-pass
filter which gives the input immunity to high frequency noise.
Figure 10.Structure of Analog Inputs
2.5 V INPUT PRECAUTIONSWhen using the 2.5 V input, the following precautions should
be noted. There is a parasitic diode between Pin 18 and VCC
due to the presence of a PMOS current source (which is used
when Pin 18 is configured as a temperature input). This will
become forward-biased if Pin 18 is more than 0.3 V above VCC.
Therefore, VCC should never be powered off with a 2.5 V input
connected.
SETTING OTHER INPUT RANGESAIN1 and AIN2 can easily be scaled to voltages other than 2.5 V.
If the input voltage range is zero to some positive voltage, all
that is required is an input attenuator, as shown in Figure 11.
Negative and bipolar input ranges can be accommodated by
using a positive reference voltage to offset the input voltage range
so it is always positive.
To measure a negative input voltage, an attenuator can be used
as shown in Figure 12.
Figure 12.Scaling and Offsetting AIN(1–2) for Negative
Inputs
This is a simple and cheap solution, but the following point
should be noted. Since the input signal is offset but not inverted,
the input range is transposed. An increase in the magnitude of
the –12 V supply (going more negative), will cause the input
voltage to fall and give a lower output code from the ADC.
Conversely, a decrease in the magnitude of the –12 V supply will
cause the ADC code to increase. The maximum negative voltage
corresponds to zero output from the ADC. This means that the
upper and lower limits will be transposed.
Bipolar input ranges can easily be accommodated. By making R1
equal to R2 and VOS = 2.5 V, the input range is ±2.5 V. Other input
ranges can be accommodated by adding a third resistor to set the
positive full-scale input voltage.
Figure 13.Scaling and Offsetting AIN(1–2) for Bipolar Inputs
(R3 has no effect as the input voltage at the device Pin is zero
when VIN = minus full-scale.)
(R2 has no effect as the input voltage at the device pin is 2.5 V
when VIN = plus full-scale).
Offset voltages other than 2.5 V can be used, but the calculation
becomes more complicated.
TEMPERATURE MEASUREMENT SYSTEM
Internal Temperature Measurement
ADM1024Register (address 4Bh). As both positive and negative tempera-
tures can be measured, the temperature data is stored in twos
complement format, as shown in Table IV. Theoretically, the
temperature sensor and ADC can measure temperatures from
–128°C to +127°C with a resolution of 1°C, although tempera-
tures below –40°C and above +125°C are outside the operating
temperature range of the device.
External Temperature MeasurementThe ADM1024 can measure the temperature of two external
diode sensors or diode-connected transistors, connected to Pins
13 and 14 or 17 and 18.
Pins 13 and 14 are a dedicated temperature input channel. Pins
17 and 18 can be configured to measure a diode sensor by set-
ting Bit 2 of the Channel Mode Register to 1.
The forward voltage of a diode or diode-connected transistor,
operated at a constant current, exhibits a negative temperature
coefficient of about –2 mV/°C. Unfortunately, the absolute value of
VBE, varies from device to device, and individual calibration is
required to null this out, so the technique is unsuitable for mass-
production.
The technique used in the ADM1024 is to measure the change
in VBE when the device is operated at two different currents.
This is given by:
∆VBE = KT/q × ln(N)
where:
K is Boltzmann’s constant
q is charge on the carrier
T is absolute temperature in Kelvins
N is ratio of the two currents.
Figure 14 shows the input signal conditioning used to measure
the output of an external temperature sensor. This figure shows the
external sensor as a substrate transistor, provided for temperature
monitoring on some microprocessors, but it could equally well
be a discrete transistor.
Figure 14.Signal Conditioning for External Diode
Temperature Sensors
If a discrete transistor is used, the collector will not be grounded,
and should be linked to the base. If a PNP transistor is used, the
base is connected to the D– input and the emitter to the D+ input.
If an NPN transistor is used, the emitter is connected to the D–
input and the base to the D+ input.
To prevent ground noise from interfering with the measurement,
the more negative terminal of the sensor is not referenced to
ground, but is biased above ground by an internal diode at the
D– input. As the sensor is operating in a noisy environment, C1 is
provided as a noise filter. See the section on layout considerations
for more information on C1.
To measure ∆VBE, the sensor is switched between operating
currents of I and N × I. The resulting waveform is passed through
a 65 kHz low-pass filter to remove noise, thence to a chopper-
stabilized amplifier that performs the functions of amplification
and rectification of the waveform to produce a dc voltage pro-
portional to ∆VBE. This voltage is measured by the ADC to give
a temperature output in 8-bit twos complement format. To
further reduce the effects of noise. Digital filtering is performed
by averaging the results of 16 measurement cycles. An external
temperature measurement takes nominally 9.6 ms.
The results of external temperature measurements are stored in
8-bit, twos-complement format, as illustrated in Table IV.
Table IV.Temperature Data Format
LAYOUT CONSIDERATIONSDigital boards can be electrically noisy environments, and care
must be taken to protect the analog inputs from noise, particularly
when measuring the very small voltages from a remote diode
sensor. The following precautions should be taken:Place the ADM1024 as close as possible to the remote sensing
diode. Provided that the worst noise sources such as clock
generators, data/address buses and CRTs are avoided, this
distance can be 4 to 8 inches.Route the D+ and D– tracks close together, in parallel, with
grounded guard tracks on each side. Provide a ground plane
under the tracks if possible.Use wide tracks to minimize inductance and reduce noise
pickup. Ten mil track minimum width and spacing is
recommended.
Figure 15.Arrangement of Signal TracksTry to minimize the number of copper/solder joints, which
can cause thermocouple effects. Where copper/solder joints
are used, make sure that they are in both the D+ and D–
path and at the same temperature.
Thermocouple effects should not be a major problem as 1°C
corresponds to about 240 µV, and thermocouple voltages are
about 3 µV/oC of temperature difference. Unless there are
two thermocouples with a big temperature differential between
them, thermocouple voltages should be much less than 200 mV.Place 0.1 µF bypass and 2200 pF input filter capacitors close
to the ADM1024.If the distance to the remote sensor is more than 8 inches, the
use of twisted pair cable is recommended. This will work up
to about 6 feet to 12 feet.For really long distances (up to 100 feet) use shielded twisted
pair such as Belden #8451 microphone cable. Connect the
twisted pair to D+ and D– and the shield to GND close to the
ADM1024. Leave the remote end of the shield unconnected
to avoid ground loops.
Because the measurement technique uses switched current
sources, excessive cable and/or filter capacitance can affect the
measurement. When using long cables, the filter capacitor may
be reduced or removed.
Cable resistance can also introduce errors. One Ω series resis-
tance introduces about 0.5°C error.
LIMIT VALUESLimit values for analog measurements are stored in the appro-
priate limit registers. In the case of voltage measurements, high
and low limits can be stored so that an interrupt request will be
generated if the measured value goes above or below acceptable
values. In the case of temperature, a Hot Temperature or High
Limit can be programmed, and a Hot Temperature Hyster-
esis or Low Limit, which will usually be some degrees lower.
This can be useful as it allows the system to be shut down when
the hot limit is exceeded, and restarted automatically when it has
cooled down to a safe temperature.
MONITORING CYCLE TIMEThe monitoring cycle begins when a one is written to the
Start Bit (Bit 0), and a zero to the INT_Clear Bit (Bit 3) of
the Configuration Register. INT_Enable (Bit 1) should be set
to one to enable the INT output. The ADC measures each analog
input in turn, as each measurement is completed the result is
As the ADC will normally be left to free-run in this manner, the
time taken to monitor all the analog inputs will normally not be
of interest, as the most recently measured value of any input can
be read out at any time.
For applications where the monitoring cycle time is important,
it can be calculated as follows:
m × t1 × n × t2
where:is the number of inputs configured as analog inputs, plus the
internal VCC measurement and internal temperature sensor.is the time taken for an analog input conversion, nominally
755 µs.is the number of inputs configured as external temperature
inputs.is the time taken for a temperature conversion, nominally
33.24 ms.
This rapid sampling of the analog inputs ensures a quick response
in the event of any input going out of limits, unlike other moni-
toring chips that employ slower ADCs.
FAN MONITORING CYCLE TIMEWhen a monitoring cycle is started, monitoring of the fan speed
inputs begins at the same time as monitoring of the analog inputs.
However, the two monitoring cycles are not synchronized in any
way. The monitoring cycle time for the fan inputs is dependent
on fan speed and is much slower than for the analog inputs. For
more details see Fan Speed Measurement section.
INPUT SAFETYScaling of the analog inputs is performed on chip, so external
attenuators are normally not required. However, since the power
supply voltages will appear directly at the pins, its is advisable to
add small external resistors in series with the supply traces to the
chip to prevent damaging the traces or power supplies should a
accidental short such as a probe connect two power supplies
together.
As the resistors will form part of the input attenuators, they will
affect the accuracy of the analog measurement if their value is
too high. The analog input channels are calibrated assuming an
external series resistor of 500 Ω, and the accuracy will remain
within specification for any value from zero to 1 kΩ, so a stan-
dard 510 Ω resistor is suitable.
The worst such accident would be connecting –12 V to +12 V—
a total of 24 V difference, with the series resistors this would draw
a maximum current of approximately 24 mA.
ANALOG OUTPUTThe ADM1024 has a single analog output from a unsigned 8-bit
DAC which produces 0 V–2.5 V. The analog output register
defaults to FF during power-on reset, which produces maximum
fan speed. The analog output may be amplified and buffered
with external circuitry such as an op amp and transistor to provide
fan speed control.
ADM1024Figure 16a.Fan Drive Circuit with Op Amp and Emitter—
Follower
Figure 16b.Fan Drive Circuit with Op Amp and PNP Transistor
Figure 16d. Discrete Fan Drive Circuit with P-Channel
MOSFET, Single Supply
12V
AOUT
4.7k�
–12VFigure 16e.Discrete Fan Drive Circuit with P-Channel
MOSFET, Dual Supply
Suitable fan drive circuits are given in Figures 16a to 16f. When
using any of these circuits, the following points should be noted:All of these circuits will provide an output range from zero
to almost 12 V, apart from Figure 17a which loses the base-
emitter voltage drop of Q1 due to the emitter-follower
configuration.To amplify the 2.5 V range of the analog output up to 12 V,
the gain of these circuits needs to be around 4.8.Care must be taken when choosing the op amp to ensure
that its input common-mode range and output voltage swing
are suitable.The op amp may be powered from the 12 V rail alone or from
±12 V. If it is powered from 12 V then the input common-
mode range should include ground to accommodate the
minimum output voltage of the DAC, and the output voltage
should swing below 0.6 V to ensure that the transistor can
be turned fully off.If the op amp is powered from –12 V, precautions such as a
clamp diode to ground may be needed to prevent the base-
emitter junction of the output transistor being reverse-biased
in the unlikely event that the output of the op amp should
swing negative for any reason.