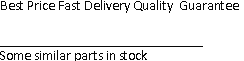
AD7825BR. ,3 V/5 V, 2 MSPS, 8-Bit, 1-, 4-, 8-Channel Sampling ADCsFEATURESFUNCTIONAL BLOCK DIAGRAM8-Bit Half-Flash ADC with 420 ns Conversion TimeV1, 4 and 8 Single- ..
AD7825BR-REEL7 ,3V/5V, 8-Bit, 4-Channel, 2 MSPS Data Acquistion SystemAPPLICATIONS*PD AD7822/AD7825Data Acquisition Systems, DSP Front Ends *V TO V AD7825/AD7829IN2 IN4* ..
AD7825BRU ,3 V/5 V, 2 MSPS, 8-Bit, 1-, 4-, 8-Channel Sampling ADCsSPECIFICATIONSV = 2.5 V. All
AD7825BRZ ,3V/5V, 8-Bit, 4-Channel, 2 MSPS Data Acquistion SystemGENERAL DESCRIPTION The AD7822 and AD7825 are available in a 20-/24-lead 0.3"The AD7822, AD7825, an ..
AD7828 ,High Speed , 8-Channel, 8-Bit CMOS ADCfeatures a per-channelconversion rate of 2.5 µs per channel, and the parts have a built-insampling ..
AD7828BQ ,LC2MOS High Speed 4- & 8-Channel 8-Bit ADCs2LC MOS High Speeda4- & 8-Channel 8-Bit ADCsAD7824/AD7828
ADM213 ,0.1 礔, +5V CMOS RS-232 200kBPS Transceiver with 4 Drivers, 5 Receivers, Shutdown and Enable Pins and 2 Receivers Active in ShutdownGENERAL DESCRIPTIONThe ADM2xx family of line drivers/receivers is intended for allSDENADM211GNDEIA- ..
ADM213AR ,0.1 uF, +5 V Powered CMOS RS-232 Drivers/ReceiversFEATURES0.1 mF to 10 mF Capacitors+5V INPUT120 kB/s Data Rate2 Receivers Active in Shutdown (ADM213 ..
ADM213AR ,0.1 uF, +5 V Powered CMOS RS-232 Drivers/Receivers0.1 mF, +5 V PoweredaCMOS RS-232 Drivers/ReceiversADM205–ADM211/ADM213TYPICAL OPERATING CIRCUIT
ADM213AR ,0.1 uF, +5 V Powered CMOS RS-232 Drivers/Receivers0.1 mF, +5 V PoweredaCMOS RS-232 Drivers/ReceiversADM205–ADM211/ADM213TYPICAL OPERATING CIRCUIT
ADM213AR-REEL , 0.1 muF, 5 V Powered CMOS RS-232 Drivers/Receivers
ADM213ARS ,0.1 uF, +5 V Powered CMOS RS-232 Drivers/ReceiversGENERAL DESCRIPTION active-high receiver enable control. Two receivers of theThe ADM2xx family of l ..
AD7822BR-AD7822BRU-AD7825BN-AD7825BR-AD7825BR.-AD7825BRU-AD7829BN-AD7829BR-AD7829BRU
3 V/5 V, 2 MSPS, 8-Bit, 1-, 4-, 8-Channel Sampling ADCs
REV.A
3 V/5 V, 2 MSPS, 8-Bit, 1-, 4-, 8-Channel
Sampling ADCs
FUNCTIONAL BLOCK DIAGRAM
FEATURES
8-Bit Half-Flash ADC with 420ns Conversion Time
1, 4 and 8 Single-Ended Analog Input Channels
Available with Input Offset Adjust
On-Chip Track-and-Hold
SNR Performance Given for Input Frequencies Up to
10 MHz
On-Chip Reference (2.5 V)
Automatic Power-Down at the End of Conversion
Wide Operating Supply Range
3 V 6 10% and 5 V 6 10%
Input Ranges
0 V to 2 V p-p, VDD = 3 V 6 10%
0 V to 2.5 V p-p, VDD = 5 V 6 10%
Flexible Parallel Interface with EOC Pulse to Allow
Stand-Alone Operation
APPLICATIONS
Data Acquisition Systems, DSP Front Ends
Disk Drives
Mobile Communication Systems, Subsampling
Applications
GENERAL DESCRIPTIONThe AD7822, AD7825, and AD7829 are high speed, 1-, 4-, and
8-channel, microprocessor-compatible, 8-bit analog-to-digital
converters with a maximum throughput of 2 MSPS. The AD7822,
AD7825, and AD7829 contain an on-chip reference of 2.5V
(2% tolerance), a track/hold amplifier, a 420 ns 8-bit half-flash
ADC and a high speed parallel interface. The converters can
operate from a single 3 V – 10% and 5 V – 10% supply.
The AD7822, AD7825, and AD7829 combine the convert start
and power-down functions at one pin, i.e., the CONVST pin.
This allows a unique automatic power-down at the end of a
conversion to be implemented. The logic level on the CONVST
pin is sampled after the end of a conversion when an EOC (End
of Conversion) signal goes high, and if it is logic low at that
point, the ADC is powered down. The AD7822 and AD7825
also have a separate power-down pin. (See Operating Modes
section of the data sheet.)
The parallel interface is designed to allow easy interfacing to
microprocessors and DSPs. Using only address decoding logic,
the parts are easily mapped into the microprocessor address
space. The EOC pulse allows the ADCs to be used in a stand-
alone manner. (See Parallel Interface section of the data sheet.)
The AD7822 and AD7825 are available in a 20-/24-lead 0.3"
wide, plastic dual-in-line package (DIP), a 20-/24-lead small
outline IC (SOIC) and a 20-/24-lead thin shrink small outline
package (TSSOP). The AD7829 is available in a 28-lead 0.6"
wide, plastic dual-in-line package (DIP), a 28-lead small outline
IC (SOIC) and in a 28-lead thin shrink small outline package
(TSSOP).
PRODUCT HIGHLIGHTSFast Conversion Time
The AD7822, AD7825, and AD7829 have a conversion time
of 420ns. Faster conversion times maximize the DSP pro-
cessing time in a real time system.Analog Input Span Adjustment
The VMID pin allows the user to offset the input span. This
feature can reduce the requirements of single supply op amps
and take into account any system offsets.FPBW (Full Power Bandwidth) of Track and Hold
The track-and-hold amplifier has an excellent high frequency
performance. The AD7822, AD7825, and AD7829 are
capable of converting full-scale input signals up to a fre-
quency of 10MHz. This makes the parts ideally suited to
subsampling applications.Channel Selection
Channel selection is made without the necessity of writing to
the part.
AD7822/AD7825/AD7829–SPECIFICATIONSLOGIC OUTPUTS
(VDD = 3 V 6 10%, VDD = 5 V 6 10%, GND = 0 V,
VREF IN/OUT = 2.5 V. All specifications –408C to +858C unless otherwise noted.)
NOTESSee Terminology section of this data sheet.Refer to the Analog Input section for an explanation of the Analog Input(s).
Specifications subject to change without notice.
ORDERING GUIDE
+2.1V
200mAIOH
OUTPUT
PIN
50pFFigure 1.Load Circuit for Access Time and Bus
Relinquish Time
AD7822/AD7825/AD7829
AD7822/AD7825/AD7829
CAUTIONESD (electrostatic discharge) sensitive device. Electrostatic charges as high as 4000V readily
accumulate on the human body and test equipment and can discharge without detection.
Although the AD7822/AD7825/AD7829 features proprietary ESD protection circuitry, perma-
nent damage may occur on devices subjected to high energy electrostatic discharges. Therefore,
proper ESD precautions are recommended to avoid performance degradation or loss of functionality.
TIMING CHARACTERISTICS1, 2t10
t11
t12
t13
tPOWER UP
NOTESSample tested to ensure compliance.See Figures 20, 21 and 22.Measured with the load circuit of Figure 1 and defined as the time required for an output to cross 0.8␣V or 2.4␣V with VDD = 5 V – 10%, and time required for an out-
put to cross 0.4␣V or 2.0␣V with VDD = 3 V – 10%.Derived from the measured time taken by the data outputs to change 0.5␣V when loaded with the circuit of Figure 1. The measured number is then extrapolated back
to remove the effects of charging or discharging the 50 pF capacitor. This means that the time, t10, quoted in the timing characteristics is the true bus relinquish time
of the part and as such is independent of external bus loading capacitances.
Specifications subject to change without notice.
ABSOLUTE MAXIMUM RATINGS*(TA = +25°C unless otherwise noted)
VDD to AGND . . . . . . . . . . . . . . . . . . . . . . . . . –0.3␣V to +7␣V
VDD to DGND . . . . . . . . . . . . . . . . . . . . . . . . . –0.3␣V to +7␣V
Analog Input Voltage to AGND
VIN1 to VIN8 . . . . . . . . . . . . . . . . . . . –0.3 V to VDD + 0.3 V
Reference Input Voltage to AGND . . . –0.3 V to VDD + 0.3␣V
VMID Input Voltage to AGND . . . . . . . –0.3 V to VDD + 0.3␣V
Digital Input Voltage to DGND . . . . . –0.3 V to VDD + 0.3 V
Digital Output Voltage to DGND . . . . –0.3 V to VDD + 0.3 V
Operating Temperature Range
Industrial (B Version) . . . . . . . . . . . . . . . . –40°C to +85°C
Storage Temperature Range . . . . . . . . . . . . –65°C to +150°C
Junction Temperature . . . . . . . . . . . . . . . . . . . . . . . . . . 150°C
Plastic DIP Package, Power Dissipation . . . . . . . . . . 450 mWJA Thermal Impedance . . . . . . . . . . . . . . . . . . . . . 105°C/W
Lead Temperature, (Soldering, 10 sec) . . . . . . . . . . . 260°C
SOIC Package, Power Dissipation . . . . . . . . . . . . . . . 450 mWJA Thermal Impedance . . . . . . . . . . . . . . . . . . . . . 75°C/W
Lead Temperature, Soldering
Vapor Phase (60 sec) . . . . . . . . . . . . . . . . . . . . . . . 215°CInfrared (15 sec) . . . . . . . . . . . . . . . . . . . . . . . . . 220°C
TSSOP Package, Power Dissipation . . . . . . . . . . . . . 450 mWJA Thermal Impedance . . . . . . . . . . . . . . . . . . . . . 128°C/W
Lead Temperature, Soldering
Vapor Phase (60 sec) . . . . . . . . . . . . . . . . . . . . . . . 215°C
Infrared (15 sec) . . . . . . . . . . . . . . . . . . . . . . . . . . . 220°C
ESD . . . . . . . . . . . . . . . . . . . . . . . . . . . . . . . . . . . . . . . . . 1 kV
*Stresses above those listed under Absolute Maximum Ratings may cause perma-
nent damage to the device. This is a stress rating only; functional operation of the
device at these or any other conditions above those listed in the operational
sections of this specification is not implied. Exposure to absolute maximum rating
conditions for extended periods may affect device reliability.
(VREF IN/OUT = 2.5 V. All specifications –408C to +858C unless otherwise noted)
PIN FUNCTION DESCRIPTIONS
PIN CONFIGURATIONS
DIP/SOIC/TSSOP
NC = NO CONNECT
DB2
DB6
DB5
DB4
DB3
DB1
DB0
CONVST
VDD
AGND
DB7CS
DGND
EOCVIN1
VMID
VREF
DB2
DB6
DB5
DB4
DB3
DB1
DB0
CONVST
VDD
AGND
DB7CS
DGND
EOCVIN1
VMID
VREF
VIN4
VIN2
VIN3
AD7822/AD7825/AD7829
TERMINOLOGY
Signal-to-(Noise + Distortion) RatioThis is the measured ratio of signal-to-(noise + distortion) at the
output of the A/D converter. The signal is the rms amplitude of
the fundamental. Noise is the rms sum of all nonfundamental
signals up to half the sampling frequency (fS/2), excluding dc.
The ratio is dependent upon the number of quantization levels
in the digitization process; the more levels, the smaller the quan-
tization noise. The theoretical signal-to-(noise + distortion) ratio
for an ideal N-bit converter with a sine wave input is given by:
Signal-to-(Noise + Distortion) = (6.02N + 1.76) dB
Thus, for an 8-bit converter, this is 50␣dB.
Total Harmonic DistortionTotal harmonic distortion (THD) is the ratio of the rms sum of
harmonics to the fundamental. For the AD7822/AD7825/AD7829
it is defined as:
where V1 is the rms amplitude of the fundamental and V2, V3,
V4, V5, and V6 are the rms amplitudes of the second through the
sixth harmonics.
Peak Harmonic or Spurious NoisePeak harmonic or spurious noise is defined as the ratio of the
rms value of the next largest component in the ADC output
spectrum (up to fS/2 and excluding dc) to the rms value of the
fundamental. Normally, the value of this specification is deter-
mined by the largest harmonic in the spectrum, but for parts
where the harmonics are buried in the noise floor, it will be a
noise peak.
Intermodulation DistortionWith inputs consisting of sine waves at two frequencies, fa and
fb, any active device with nonlinearities will create distortion
products at sum and difference frequencies of mfa – nfb where
m, n = 0, 1, 2, 3, etc. Intermodulation terms are those for which
neither m nor n are equal to zero. For example, the second order
terms include (fa + fb) and (fa – fb), while the third order terms
include (2fa + fb), (2fa – fb), (fa + 2fb) and (fa – 2fb).
The AD7822/AD7825/AD7829 are tested using the CCIF stan-
dard where two input frequencies near the top end of the input
bandwidth are used. In this case, the second and third order
terms are of different significance. The second order terms are
usually distanced in frequency from the original sine waves
while the third order terms are usually at a frequency close to
the input frequencies. As a result, the second and third order
terms are specified separately. The calculation of the intermodula-
tion distortion is as per the THD specification where it is the
ratio of the rms sum of the individual distortion products to the
rms amplitude of the fundamental expressed in dBs.
Channel-to-Channel IsolationChannel-to-channel isolation is a measure of the level of crosstalk
between channels. It is measured by applying a full-scale 20kHz
sine wave signal to one input channel and determining how
much that signal is attenuated in each of the other channels.
Relative AccuracyRelative accuracy or endpoint nonlinearity is the maximum
deviation from a straight line passing through the endpoints of
the ADC transfer function.
Differential NonlinearityThe difference between the measured and the ideal 1LSB change
between any two adjacent codes in the ADC.
Offset ErrorThe deviation of the 128th code transition (01111111) to
(10000000) from the ideal, i.e., VMID.
Offset Error MatchThe difference in offset error between any two channels.
Zero-Scale ErrorThe deviation of the first code transition (00000000) to
(00000001) from the ideal, i.e., VMID – 1.25 V + 1 LSB (VDD =
5 V – 10%), or VMID – 1.0 V + 1 LSB (VDD = 3 V – 10%).
Full-Scale ErrorThe deviation of the last code transition (11111110) to
(11111111) from the ideal, i.e., VMID + 1.25 V – 1 LSB (VDD =
5 V – 10%), or VMID + 1.0 V – 1 LSB (VDD = 3 V – 10%).
Gain ErrorThe deviation of the last code transition (1111...110) to
(1111...111) from the ideal, i.e., VREF – 1 LSB, after the off-
set error has been adjusted out.
Gain Error MatchThe difference in gain error between any two channels.
Track/Hold Acquisition TimeThe time required for the output of the track/hold amplifier to
reach its final value, within –1/2 LSB, after the point at which
the track/hold returns to track mode. This happens approxi-
mately 120 ns after the falling edge of CONVST.
It also applies to situations where a change in the selected input
channel takes place or where there is a step input change on the
input voltage applied to the selected VIN input of the AD7822/
AD7825/AD7829. It means that the user must wait for the dura-
tion of the track/hold acquisition time after a channel change/step
input change to VIN before starting another conversion, to
ensure that the part operates to specification.
PSR (Power Supply Rejection)Variations in power supply will affect the full-scale transition,
but not the converter’s linearity. Power supply rejection is the
maximum change in the full-scale transition point due to a
change in power supply voltage from the nominal value.
CIRCUIT DESCRIPTIONThe AD7822, AD7825, and AD7829 consist of a track-and-hold
amplifier followed by a half-flash analog-to-digital converter.
These devices use a half-flash conversion technique where one
4-bit flash ADC is used to achieve an 8-bit result. The 4-bit
flash ADC contains a sampling capacitor followed by fifteen
comparators that compare the unknown input to a reference
ladder to achieve a 4-bit result. This first flash, i.e., coarse con-
version, provides the 4 MSBs. For a full 8-bit reading to be
realized, a second flash, i.e., a fine conversion, must be per-
Figures 2 and 3 below show simplified schematics of the ADC.
When the ADC starts a conversion, the track and hold goes into
hold mode and holds the analog input for 120 ns. This is the
acquisition phase as shown in Figure 2, when Switch 2 is in
Position A. At the point when the track and hold returns to
its track mode, this signal is sampled by the sampling capacitor
as Switch 2 moves into Position B. The first flash occurs at this
instant and is then followed by the second flash. Typically, the
first flash is complete after 100 ns, i.e., at 220 ns, while the end
of the second flash and hence the 8-bit conversion result is
available at 330 ns. As shown in Figure 4, the track-and-hold
returns to track mode after 120 ns, and starts the next acquisi-
tion before the end of the current conversion. Figure 6 shows
the ADC transfer function.
VINFigure 2.ADC Acquisition Phase
VINFigure 4.Track-and-Hold Timing
TYPICAL CONNECTION DIAGRAMFigure 5 shows a typical connection diagram for the AD7822,
AD7825, and AD7829. The AGND and DGND are connected
together at the device for good noise suppression. The parallel
interface is implemented using an 8-bit data bus. The end of
conversion signal (EOC) idles high, the falling edge of CONVST
initiates a conversion and at the end of conversion the falling
edge of EOC is used to initiate an Interrupt Service Routine
(ISR) on a microprocessor. (See Parallel interface section for
more details.) VREF and VMID are connected to voltage source
such as the AD780, while VDD is connected to a voltage source
that can vary from 4.5 V to 5.5 V. (See Table I in Analog Input
section.) When VDD is first connected, the AD7822, AD7825, and
AD7829 power up in a low current mode, i.e., power-down, with
the default logic level on the EOC pin on the AD7822 and
AD7825 equal to a low. Ensure the CONVST line is not floating
when VDD is applied, as this could put the AD7822/AD7825/
AD7829 into an unknown state. A rising edge on the CONVST
pin will cause the AD7829 to fully power up while a rising edge
on the PD pin will cause the AD7822 and AD7825 to fully power
up. For applications where power consumption is of concern,
the automatic power-down at the end of a conversion should be
used to improve power performance. (See Power-Down Options
section of the data sheet.)
Figure 5.Typical Connection Diagram
AD7822/AD7825/AD7829
ADC TRANSFER FUNCTIONThe output coding of the AD7822, AD7825, and AD7829 is
straight binary. The designed code transitions occur at succes-
sive integer LSB values (i.e., 1 LSB, 2 LSBs, etc.). The LSB size
is = VREF/256 (VDD = 5 V) or the LSB size = (0.8 VREF)/256
(VDD = 3 V). The ideal transfer characteristic for the AD7822,
AD7825, and AD7829 is shown in Figure 6, below.
ADC CODE
(VDD = 5V) VMID–1.25V
(VDD = 3V) VMID–1V
VMID+1.25V–1LSB
VMID+1V–1LSB
ANALOG INPUT VOLTAGEFigure 6.Transfer Characteristic
ANALOG INPUTThe AD7822 has a single input channel and the AD7825 and
AD7829 have four and eight input channels respectively. Each
input channel has an input span of 2.5 V or 2.0 V, depending on
the supply voltage (VDD). This input span is automatically set
up by an on-chip “VDD Detector” circuit. 5 V operation of the
ADCs is detected when VDD exceeds 4.1 V and 3 V operation is
detected when VDD falls below 3.8 V. This circuit also possesses
a degree of glitch rejection; for example, a glitch from 5.5 V to
2.7 V up to 60 ns wide will not trip the VDD detector.
The VMID pin is used to center this input span anywhere in the
range AGND to VDD. If no input voltage is applied to VMID, i.e.,
if VMID is left unconnected, the default input range is AGND
to 2.0 V (VDD = 3 V – 10%) i.e., centered about 1.0 V, or
AGND to 2.5 V (VDD = 5 V – 10%) i.e., centered about 1.25V.
If, however, an external VMID is applied, the analog input range
will be from VMID – 1.0 V to VMID + 1.0 V (VDD = 3 V – 10%),
or from VMID – 1.25 V to VMID + 1.25 V (VDD = 5 V – 10%).
The range of values of VMID that can be applied depends on the
value of VDD. For VDD = 3 V – 10%, the range of values that
can be applied to VMID is from 1.0 V to VDD – 1.0 V and is 1.25 V
to VDD – 1.25 V when VDD= 5 V – 10%. Table I shows the rel-
evant ranges of VMID and the input span for various values of
VDD. Figure 7 illustrates the input signal range available with
various values of VMID.
Table I.Figure 7.Analog Input Span Variation with VMID
VMID may be used to remove offsets in a system by applying the
offset to the VMID pin as shown in Figure 8, or it may be used to
accommodate bipolar signals by applying VMID to a level-shifting
circuit before VIN, as shown in Figure 9. When VMID is being
driven by an external source, the source may be directly tied to
the level-shifting circuitry, see Figure 9; however, if the internal
VMID, i.e., the default value, is being used as an output, it must
be buffered before applying it to the level-shifting circuitry
as the VMID pin has an impedance of approximately 6kW, see
Figure 10.
VIN
VMID
VMID
VIN
VMIDFigure 8.Removing Offsets Using VMID
Figure 9.Accommodating Bipolar Signals Using
External VMID
V
VIN
VMIDFigure 10.Accommodating Bipolar Signals Using
Internal VMID
NOTE: Although there is a VREF pin from which a voltage
reference of 2.5 V may be sourced, or to which an external ref-
erence may be applied, this does not provide an option of
varying the value of the voltage reference. As stated in the
specifications for the AD7822, AD7825, and AD7829, the input
voltage range at this pin is 2.5 V – 2%.
Analog Input StructureFigure 11 shows an equivalent circuit of the analog input structure
of the AD7822, AD7825, and the AD7829. The two diodes, D1
and D2, provide ESD protection for the analog inputs. Care
must be taken to ensure that the analog input signal never exceeds
the supply rails by more than 200 mV. This will cause these
diodes to become forward biased and start conducting current into
the substrate. 20 mA is the maximum current these diodes can
conduct without causing irreversible damage to the part. How-
ever, it is worth noting that a small amount of current (1 mA)
being conducted into the substrate due to an over voltage on an
unselected channel, can cause inaccurate conversions on a se-
lected channel. The capacitor C2 in Figure 11 is typically about
4 pF and can be primarily attributed to pin capacitance. The
resistor, R1, is a lumped component made up of the on resis-
tance of several components, including that of the multiplexer
and the track and hold. This resistor is typically about 310 W.
The capacitor C1 is the track-and-hold capacitor and has a ca-
pacitance of 0.5 pF. Switch 1 is the track-and-hold switch, while
Switch 2 is that of the sampling capacitor as shown in Figures
2 and 3.
When in track phase, Switch 1 is closed and Switch 2 is in
Position A; when in hold mode, Switch 1 opens while Switch
2 remains in Position A. The track-and-hold remains in hold
mode for 120 ns—see Circuit Description, after which it returns
to track mode and the ADC enters its conversion phase. At this
point Switch 1 opens and Switch 2 moves to Position B. At the
end of the conversion Switch 2 moves back to Position A.
Figure 11.Equivalent Analog Input Circuit
Analog Input SelectionOn power-up, the default VIN selection is VIN1. When returning
to normal operation from power-down, the VIN selected will be
the same one that was selected prior to power-down being initiated.
Table II below shows the multiplexer address corresponding to
each analog input from VIN1 to VIN4(8) for the AD7825 or AD7829.
Table II.Channel selection on the AD7825 and AD7829 is made without
the necessity of a write operation. The address of the next channel
to be converted is latched at the start of the current read operation,
as shown in Figure 12. This allows for improved throughput rates
in “channel hopping” applications.