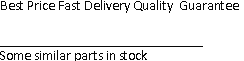
AD7711AAR ,LC2MOS Signal Conditioning ADC with RTD Current Sourceapplications with an on-chip control register that allowsThe AD7711A is ideal for use in smart, mic ..
AD7711AN ,LC2MOS Signal Conditioning ADC with RTD Excitation Currentsapplications. The device accepts low level signalsoptions and also allows the user to read and writ ..
AD7711ANZ , LC2MOS Signal Conditioning ADC with RTD Excitation Currents
AD7711AR ,LC2MOS Signal Conditioning ADC with RTD Excitation Currentsfeatures one differential analog input and one singlePRODUCT HIGHLIGHTSended analog input as well a ..
AD7712AN ,LC2MOS Signal Conditioning ADCspecifications T to T unless otherwise noted.)MIN MAX1Parameter A, S Versions Units Conditions/Comm ..
AD7712AQ ,LC2MOS Signal Conditioning ADCFEATURESFUNCTIONAL BLOCK DIAGRAMCharge Balancing ADCREF REFDV V24 Bits No Missing Codes AVDD DD IN ..
ADM1031ARQ-REEL7 ,Complete, ACPI Compliant, Dual Channel ±1°C Remote Thermal Monitor with Integrated Fan Controller for two Independent Fans, ...SPECIFICATIONS A MIN MAX CC MIN MAXParameter Min Typ Max Unit Test Conditions/CommentsPOWER SUPPLYS ..
ADM1031ARQZ ,Complete, ACPI Compliant, Dual Channel ±1°C Remote Thermal Monitor with Integrated Fan Controller for two Independent Fans, ...FEATURES PRODUCT DESCRIPTION®Optimized for Pentium III: Allows Reduced Guardbanding The ADM1031 is ..
ADM1031ARQZ-R7 , Intelligent Temperature Monitor and Dual PWM Fan Controller
ADM1031ARQZ-REEL ,Complete, ACPI Compliant, Dual Channel ±1°C Remote Thermal Monitor with Integrated Fan Controller for two Independent Fans, ...Specifications subject to change without notice.–2– REV. AADM1031ABSOLUTE MAXIMUM RATINGS* ORDERING ..
ADM1032AR ,+-1C Remote and Local System Temperature MonitorSPECIFICATIONS (T = T to T , V = V to V , unless otherwise noted.)A MIN MAX DD MIN MAXParameter Min ..
ADM1032AR-1 ,High Accuracy, Remote Thermal Diode Monitor in Micro SOIC PackageSPECIFICATIONSParameter Min Typ Max Unit Test Conditions/CommentsPOWER SUPPLYSupply Voltage, V 3.0 ..
AD7711AAR
LC2MOS Signal Conditioning ADC with RTD Current Source
FUNCTIONAL BLOCK DIAGRAM
AGNDDGNDMODESDATASCLKA0
MCLK
OUT
MCLK
AIN1(+)
AIN1(–)
REF
IN (–)
REF
IN (+)
SYNC
DRDYTFSRFS
REF OUT
AIN2(+)
AIN2(–)
VSS
VBIASAVDDDVDD
RTD
CURRENT
FEATURES
Charge Balancing ADC
24 Bits No Missing Codes60.0015% Nonlinearity
Two-Channel Programmable Gain Front End
Gains from 1 to 128
Differential Inputs
Low-Pass Filter with Programmable Filter Cutoffs
Ability to Read/Write Calibration Coefficients
Bidirectional Microcontroller Serial Interface
Internal/External Reference Option
Single or Dual Supply Operation
Low Power (25 mW typ) with Power-Down Mode
(7 mW typ)
APPLICATIONS
RTD Transducers2MOS Signal Conditioning ADC
with RTD Current SourceREV.C
GENERAL DESCRIPTIONThe AD7711A is a complete analog front end for low frequency
measurement applications. The device accepts low level signals
directly from a transducer and outputs a serial digital word. It
employs a sigma-delta conversion technique to realize up to
24 bits of no missing codes performance. The input signal is
applied to a proprietary programmable gain front end based
around an analog modulator. The modulator output is pro-
cessed by an on-chip digital filter. The first notch of this digital
filter can be programmed via the on-chip control register allow-
ing adjustment of the filter cutoff and settling time.
The part features two differential analog inputs and a differen-
tial reference input. Normally, one of the channels will be used
as the main channel with the second channel used as an auxil-
iary input to periodically measure a second voltage. It can be
operated from a single supply (by tying the VSS pin to AGND)
provided that the input signals on the analog inputs are more
positive than –30 mV. By taking the VSS pin negative, the part
can convert signals down to –VREF on its inputs. The part also
provides a 400 mA current source that can be used to provide
excitation for RTD transducers. The AD7711A thus performs
all signal conditioning and conversion for a single or dual chan-
nel system.
The AD7711A is ideal for use in smart, microcontroller-based
systems. Input channel selection, gain settings and signal polar-
ity can be configured in software using the bidirectional serial
port. The AD7711A contains self-calibration, system calibration
and background calibration options and also allows the user to
read and write the on-chip calibration registers.
*Protected by U.S. Patent No. 5,134,401.CMOS construction ensures low power dissipation, and a soft-
ware programmable power-down mode reduces the standby
power consumption to only 7 mW typical. The part is available
in a 24-lead, 0.3 inch-wide, hermetic dual-in-line package
(cerdip) as well as a 24-lead small outline (SOIC) package.
PRODUCT HIGHLIGHTSThe programmable gain front end allows the AD7711A
to accept input signals directly from an RTD transducer,
removing a considerable amount of signal conditioning. An
on-chip current source provides the excitation current for
the RTD.The part features excellent static performance specifications
with 24-bit no missing codes, –0.0015% accuracy and low
rms noise (<250 nV). Endpoint errors and the effects of
temperature drift are eliminated by on-chip calibration op-
tions, which remove zero-scale and full-scale errors.The AD7711A is ideal for microcontroller or DSP processor
applications with an on-chip control register that allows
control over filter cutoff, input gain, channel selection, signal
polarity, RTD current control and calibration modes.The AD7711A allows the user to read and to write the
on-chip calibration registers. This means that the micro-
controller has much greater control over the calibration
procedure.
NOTESTemperature ranges are as follows: A Version, –40°C to +85°C; S Version, –55°C to +125°C.Applies after calibration at the temperature of interest.Positive full-scale error applies to both unipolar and bipolar input ranges.These errors will be of the order of the output noise of the part as shown in Table I when using system calibration. These errors are 20 mV typical when using self-
calibration or background calibration.Recalibration at any temperature or use of the background calibration mode will remove these drift errors.This common-mode voltage range is allowed provided that the input voltage on AIN(+) and AIN(–) does not exceed AVDD + 30 mV and VSS – 30 mV.These numbers are guaranteed by design and/or characterization.The analog inputs present a very high impedance dynamic load which varies with clock frequency and input sample rate. The maximum recommended source
resistance depends on the selected gain (see Tables IV and V).The analog input voltage range on the AIN1(+) and AIN2(+) inputs is given here with respect to the voltage on the AIN1(–) and AIN2(–) inputs. The absolute
voltage on the analog inputs should not go more positive than AVDD + 30 mV or go more negative than VSS – 30 mV.VREF = REF IN(+) – REF IN(–).The reference input voltage range may be restricted by the input voltage range requirement on the VBIAS input.
AD7711A–SPECIFICATIONS(AVDD = +5␣V 6 5%; DVDD = +5␣V 6 5%; VSS = 0 V or –5␣V 6 5%; REF IN(+) = +2.5␣V;
REF␣IN(–) = AGND; MCLK IN = 10␣MHz unless otherwise stated. All specifications TMIN to TMAX unless otherwise noted.)
LOGIC OUTPUTS
SYSTEM CALIBRATION
AD7711A
NOTESThe AD7711A is specified with a 10 MHz clock for AVDD voltages of +5 V – 5%. It is specified with an 8 MHz clock for AVDD voltages greater than 5.25 V and less
than 10.5 V.The –5% tolerance on the DVDD input is allowed provided that DVDD does not exceed AVDD by more than 0.3 V.Measured at dc and applies in the selected passband. PSRR at 50 Hz will exceed 120 dB with filter notches of 10 Hz, 25 Hz or 50 Hz. PSRR at 60 Hz will exceed
120 dB with filter notches of 10 Hz, 30 Hz or 60 Hz.PSRR depends on gain: Gain of 1: 70 dB typ; Gain of 2: 75 dB typ; Gain of 4: 80 dB typ; Gains of 8 to 128: 85 dB typ. These numbers can be improved (to 95 dB
typ) by deriving the VBIAS voltage (via Zener diode or reference) from the AVDD supply.
Specifications subject to change without notice.
AD7711A–SPECIFICATIONS
ABSOLUTE MAXIMUM RATINGS*(TA = +25°C, unless otherwise noted)
AVDD to DVDD . . . . . . . . . . . . . . . . . . . . . . . –0.3 V to +12 V
AVDD to VSS . . . . . . . . . . . . . . . . . . . . . . . . . . .–0.3 V to +12 V
AVDD to AGND . . . . . . . . . . . . . . . . . . . . . . –0.3 V to +12 V
AVDD to DGND . . . . . . . . . . . . . . . . . . . . . . –0.3 V to +12 V
DVDD to AGND . . . . . . . . . . . . . . . . . . . . . . . –0.3 V to +6 V
DVDD to DGND . . . . . . . . . . . . . . . . . . . . . . . –0.3 V to +6 V
VSS to AGND . . . . . . . . . . . . . . . . . . . . . . . . . +0.3 V to –6 V
VSS to DGND . . . . . . . . . . . . . . . . . . . . . . . . . +0.3 V to –6 V
Analog Input Voltage to AGND
. . . . . . . . . . . . . . . . . . . . . . . . . VSS – 0.3 V to AVDD + 0.3 V
Reference Input Voltage to AGND
. . . . . . . . . . . . . . . . . . . . . . . . . VSS – 0.3 V to AVDD + 0.3 V
REF OUT to AGND . . . . . . . . . . . . . . . . . . . .–0.3 V to AVDD
Digital Input Voltage to DGND . . . . .–0.3 V to AVDD + 0.3 V
Digital Output Voltage to DGND . . . –0.3 V to DVDD + 0.3 V
Operating Temperature Range
Commercial (A Version) . . . . . . . . . . . . . . .–40°C to +85°C
Extended (S Version) . . . . . . . . . . . . . . . . .–55°C to +125°C
Storage Temperature Range . . . . . . . . . . . . .–65°C to +150°C
Lead Temperature (Soldering, 10 secs) . . . . . . . . . . . .+300°C
Power Dissipation (Any Package) to +75°C . . . . . . . .450 mW
Derates Above +75°C . . . . . . . . . . . . . . . . . . . . . . . .6 mW/°C
*Stresses above those listed under Absolute Maximum Ratings may cause perma-
nent damage to the device. This is a stress rating only; functional operation of the
device at these or any other conditions above those listed in the operational
sections of the specification is not implied. Exposure to absolute maximum rating
conditions for extended periods may affect device reliability.
ORDERING GUIDE*R = SOIC, Q = Cerdip.
CAUTIONESD (electrostatic discharge) sensitive device. Electrostatic charges as high as 4000V readily
accumulate on the human body and test equipment and can discharge without detection.
TIMING CHARACTERISTICS1, 2(DVDD = +5␣V 6 5%; AVDD = +5␣V or +10␣V3, 6 5%; VSS = 0 V or –5 V 6 10%; AGND = DGND
= 0 V; fCLKIN = 10␣MHz; Input Logic 0 = 0 V, Logic 1 = DVDD unless otherwise noted)AD7711ANOTESGuaranteed by design, not production tested. All input signals are specified with tr = tf = 5 ns (10% to 90% of 5 V) and timed from a voltage level of 1.6 V.See Figures 10 to 13.The AD7711A is specified with a 10 MHz clock for AVDD voltages of +5 V – 5%. It is specified with an 8 MHz clock for AVDD voltages greater than 5.25 V and less
than 10.5 V.CLK IN duty cycle range is 45% to 55%. CLK IN must be supplied whenever the AD7711A is not in STANDBY mode. If no clock is present in this case, the device
can draw higher current than specified and possibly become uncalibrated.The AD7711A is production tested with fCLK IN at 10 MHz (8 MHz for AVDD > +5.25 V). It is guaranteed by characterization to operate at 400 kHz.Specified using 10% and 90% points on waveform of interest.These numbers are measured with the load circuit of Figure 1 and defined as the time required for the output to cross 0.8 V or 2.4 V.These numbers are derived from the measured time taken by the data output to change 0.5 V when loaded with the circuit of Figure 1. The measured number is then
extrapolated back to remove effects of charging or discharging the 100 pF capacitor. This means that the times quoted in the timing characteristics are the true bus
relinquish times of the part and as such are independent of external bus loading capacitances.
Specifications subject to change without notice.
Figure 1. Load Circuit for Access Time and Bus Relinquish
Time
PIN CONFIGURATION
DIP and SOIC
AVDD
VSS
AIN1(–)
SCLK
MCLK IN
MCLK OUT
AIN1(+)
MODE
SYNC
VBIAS
REF IN(–)
REF IN(+)
REF OUT
RTD CURRENT
DGND
DVDD
SDATA
DRDY
AGND
TFS
RFS
AIN2(–)
AIN2(+)
PIN FUNCTION DESCRIPTIONSAD7711A
TERMINOLOGY
INTEGRAL NONLINEARITYThis is the maximum deviation of any code from a straight line
passingthroughtheendpointsofthetransferfunction. The end-
points of the transfer function are zero-scale (not to be confused
withbipolarzero),apoint0.5LSBbelowthefirstcode transi-
tion (000...000 to 000...001) and full scale, a point 0.5LSB
abovethelastcodetransition(111...110to 111...111). The
error is expressed as a percentage of full scale.
POSITIVE FULL-SCALE ERRORPositive Full-Scale Error is the deviation of the last code transi-
tion (111...110 to 111...111) from the ideal AIN(+) voltage
(AIN(–) + VREF/GAIN – 3/2 LSBs). It applies to both unipolar
and bipolar analog input ranges.
UNIPOLAR OFFSET ERRORUnipolar Offset Error is the deviation of the first code transition
from the ideal AIN(+) voltage (AIN(–) + 0.5 LSB) when oper-
ating in the unipolar mode.
BIPOLAR ZERO ERRORThis is the deviation of the midscale transition (0111...111
to 1000...000) fromtheidealAIN(+)voltage(AIN(–)
– 0.5LSB) when operating in the bipolar mode.
BIPOLAR NEGATIVE FULL-SCALE ERRORThis is the deviation of the first code transition from the ideal
AIN(+) voltage (AIN(–) – VREF/GAIN + 0.5␣LSB), when oper-
ating in the bipolar mode.
POSITIVE FULL-SCALE OVERRANGEPositive Full-Scale Overrange is the amount of overhead avail-
able to handle input voltages on AIN(+) input greater than
AIN(–) + VREF/GAIN (for example, noise peaks or excess volt-
ages due to system gain errors in system calibration routines)
without introducing errors due to overloading the analog modu-
lator or overflowing the digital filter.
NEGATIVE FULL-SCALE OVERRANGEThis is the amount of overhead available to handle voltages on
AIN(+) below AIN(–) – VREF/GAIN without overloading the
analog modulator or overflowing the digital filter. Note that the
analog input will accept negative voltage peaks even in the uni-
polar mode provided that AIN(+) is greater than AIN(–) and
greater than VSS – 30␣mV.
OFFSET CALIBRATION RANGEIn the system calibration modes, the AD7711A calibrates its
offset with respect to the analog input. The Offset Calibration
Range specification defines the range of voltages that the
AD7711A can accept and still accurately calibrate offset.
FULL-SCALE CALIBRATION RANGEThis is the range of voltages that the AD7711A can accept in
the system calibration mode and still correctly calibrate full-scale.
INPUT SPANIn system calibration schemes, two voltages applied in sequence
to the AD7711A’s analog input define the analog input range.
The input span specification defines the minimum and maxi-
mum input voltages from zero to full-scale that the AD7711A
can accept and still calibrate accurately gain.
CONTROL REGISTER (24 BITS)A write to the device with the A0 input low writes data to the control register. A read to the device with the A0 input low accesses the
contents of the control register. The control register is 24 bits wide and when writing to the register 24 bits of data must be written
otherwise the data will not be loaded to the control register. In other words, it is not possible to write just the first 12 bits of data into
the control register. If more than 24 clock pulses are provided before TFS returns high, then all clock pulses after the 24th clock
pulse are ignored. Similarly, a read operation from the control register should access 24 bits of data.
MSB
LSB *Must always be 0 to ensure correct operation of the device.
AD7711A
PGA GAINGlG0Gain0001(Default Condition After the Internal Power-On Reset)
Channel SelectionChannelAIN1(Default Condition After the Internal Power-On Reset)AIN2
Power-DownNormal Operation(Default Condition After the Internal Power-On Reset)Power-Down
Word LengthOutput Word Length16-Bit(Default Condition After Internal Power-On Reset)24-Bit
RTD Excitation CurrentOff(Default Condition After Internal Power-On Reset)
1On
Burnout CurrentOff(Default Condition After Internal Power-On Reset)
1On
Bipolar/Unipolar Selection (Both Inputs)
B/U0 Bipolar(Default Condition After Internal Power-On Reset)
1 Unipolar
Filter Selection (FS11–FS0)The on-chip digital filter provides a Sinc3 (or (Sinx/x)3) filter response. The 12 bits of data programmed into these bits determine
the filter cutoff frequency, the position of the first notch of the filter and the data rate for the part. In association with the gain selec-
tion, it also determines the output noise (and hence the effective resolution) of the device.
The first notch of the filter occurs at a frequency determined by the relationship: filter first notch frequency = (fCLK IN/512)/code
where code is the decimal equivalent of the code in bits FS0 to FS11 and is in the range 19 to 2,000. With the nominal fCLK IN of
10 MHz, this results in a first notch frequency range from 9.76 Hz to 1.028 kHz. To ensure correct operation of the AD7711A, the
value of the code loaded to these bits must be within this range. Failure to do this will result in unspecified operation of the device.
Changing the filter notch frequency, as well as the selected gain, impacts resolution. Tables I and II and Figure 2 show the effect of
the filter notch frequency and gain on the effective resolution of the AD7711A. The output data rate (or effective conversion time)
for the device is equal to the frequency selected for the first notch of the filter. For example, if the first notch of the filter is selected
at 50 Hz, then a new word is available at a 50 Hz rate or every 20 ms. If the first notch is at 1 kHz, a new word is available every 1 ms.
The settling time of the filter to a full-scale step input change is worst case 4 · 1/(output data rate). This settling time is to 100% of
the final value. For example, with the first filter notch at 50 Hz, the settling time of the filter to a full-scale step input change is
80 ms max. If the first notch is at 1 kHz, the settling time of the filter to a full-scale input step is 4 ms max. This settling time can be
reduced to 3 · l/(output data rate) by synchronizing the step input change to a reset of the digital filter. In other words, if the step
input takes place with SYNC low, the settling time will be 3 · l/(output data rate). If a change of channels takes place, the settling
time is 3 · l/(output data rate) regardless of the SYNC input.
The –3 dB frequency is determined by the programmed first notch frequency according to the relationship: filter –3 dB frequency
Tables I and II show the output rms noise for some typical notch and –3 dB frequencies. The numbers given are for the bipolar
input ranges with a VREF of +2.5 V. These numbers are typical and are generated with an analog input voltage of 0 V. The output
noise from the part comes from two sources. First, there is the electrical noise in the semiconductor devices used in the implementa-
tion of the modulator (device noise). Secondly, when the analog input signal is converted into the digital domain, quantization noise
is added. The device noise is at a low level and is largely independent of frequency. The quantization noise starts at an even lower
level but rises rapidly with increasing frequency to become the dominant noise source. Consequently, lower filter notch settings
(below 60 Hz approximately) tend to be device noise dominated while higher notch settings are dominated by quantization noise.
Changing the filter notch and cutoff frequency in the quantization noise dominated region results in a more dramatic improvement
in noise performance than it does in the device noise dominated region as shown in Table I. Furthermore, quantization noise is
added after the PGA, so effective resolution is independent of gain for the higher filter notch frequencies. Meanwhile, device noise is
added in the PGA and, therefore, effective resolution suffers a little at high gains for lower notch frequencies.
At the lower filter notch settings (below 60 Hz), the no missing codes performance of the device is at the 24-bit level. At the higher
settings, more codes will be missed until at 1 kHz notch setting, no missing codes performance is only guaranteed to the 12-bit level.
However, since the effective resolution of the part is 10.5 bits for this filter notch setting, this no missing codes performance should
be more than adequate for all applications.
The effective resolution of the device is defined as the ratio of the output rms noise to the input full scale. This does not remain
constant with increasing gain or with increasing bandwidth. Table II shows the same table as Table I except that the output is now
expressed in terms of effective resolution (the magnitude of the rms noise with respect to 2 · VREF/GAIN, i.e., the input full scale). It
is possible to do post filtering on the device to improve the output data rate for a given –3 dB frequency and also to further reduce
the output noise (see Digital Filtering section).
Table I.Output Noise vs. Gain and First Notch Frequency10␣
NOTESThe default condition (after the internal power-on reset) for the first notch of filter is 60 Hz.For these filter notch frequencies, the output rms noise is primarily dominated by device noise and as a result is independent of the value of the reference voltage.
Therefore, increasing the reference voltage will give an increase in the effective resolution of the device (i.e., the ratio of the rms noise to the input full scale is in-
creased since the output rms noise remains constant as the input full-scale increases).For these filter notch frequencies, the output rms noise is dominated by quantization noise and as a result is proportional to the value of the reference voltage.
Table II.Effective Resolution vs. Gain and First Notch Frequency
Filter & O/PNOTEEffective resolution is defined as the magnitude of the output rms noise with respect to the input full scale (i.e., 2 · VREF/GAIN). The above table applies for a VREF
of +2.5 V and resolution numbers are rounded to the nearest 0.5 LSB.
AD7711AFigures 2a and 2b give information similar to that outlined in Table I. In these plots, the output rms noise is shown for the full
range of available cutoffs frequencies rather than for some typical cutoff frequencies as in Tables I and II. The numbers given in
these plots are typical values at +25°C.
Figure 2a.Plot of Output Noise vs. Gain and Notch
Frequency (Gains of 1 to 8)
CIRCUIT DESCRIPTIONThe AD7711A is a sigma-delta A/D converter with on-chip
digital filtering, intended for the measurement of wide dynamic
range, low frequency signals such as those in weigh scale, indus-
trial control or process control applications. It contains a sigma-
delta (or charge balancing) ADC, a calibration microcontroller
with on-chip static RAM, a clock oscillator, a digital filter and a
bidirectional serial communications port.
The part contains two programmable gain differential analog
input channels. The gain range is from 1 to 128 allowing the
part to accept unipolar signals of between 0 mV to +20 mV and
0 V to +2.5 V or bipolar signals in the range from –20 mV to2.5 V when the reference input voltage equals +2.5 V. The
input signal to the selected analog input channel is continuously
sampled at a rate determined by the frequency of the master
clock, MCLK IN, and the selected gain (see Table III). A
charge balancing A/D converter (Sigma-Delta Modulator) con-
verts the sampled signal into a digital pulse train whose duty
cycle contains the digital information. The programmable gain
function on the analog input is also incorporated in this sigma-
delta modulator with the input sampling frequency being modi-
fied to give the higher gains. A sinc3 digital low-pass filter
processes the output of the sigma-delta modulator and updates
the output register at a rate determined by the first notch fre-
quency of this filter. The output data can be read from the serial
port randomly or periodically at any rate up to the output regis-
ter update rate. The first notch of this digital filter (and hence
its –3 dB frequency) can be programmed via an on-chip control
register. The programmable range for this first notch frequency
is from 9.76 Hz to 1.028 kHz, giving a programmable range for
the –3 dB frequency of 2.58 Hz to 269 Hz.
Figure 2b.Plot of Output Noise vs. Gain and Notch
Frequency (Gains of 16 to 128)
The basic connection diagram for the part is shown in Figure 3.
This shows the AD7711A in the external clocking mode with
both the AVDD and DVDD pins of the AD7711A being driven
from the analog +5 V supply. Some applications will have
separate supplies for both AVDD and DVDD, and in some of
these cases, the analog supply will exceed the +5 V digital sup-
ply (see Power Supplies and Grounding section).
Figure 3.Basic Connection Diagram
The AD7711A provides a number of calibration options which
can be programmed via the on-chip control register. A calibra-
tion cycle may be initiated at any time by writing to this control
register. The part can perform self-calibration using the on-chip
calibration microcontroller and SRAM to store calibration pa-
rameters. Other system components may also be included in the
calibration loop to remove offset and gain errors in the input
channel using the system calibration mode. Another option is a
background calibration mode where the part continuously per-
forms self-calibration and updates the calibration coefficients.
Once the part is in this mode, the user does not have to worry
about issuing periodic calibration commands to the device or
ask the device to recalibrate when there is a change in the ambi-
ent temperature or power supply voltage.
The AD7711A gives the user access to the on-chip calibration
registers allowing the microprocessor to read the device’s cali-
bration coefficients and also to write its own calibration coeffi-
cients to the part from prestored values in E2PROM. This gives
the microprocessor much greater control over the AD7711A’s
calibration procedure. It also means that the user can verify that
the device has performed its calibration correctly by comparing the
coefficients after calibration with prestored values in E2PROM.
The AD7711A can be operated in single supply systems pro-
vided that the analog input voltage does not go more negative
than –30 mV. For larger bipolar signals, a VSS of –5 V is re-
quired by the part. For battery operation, the AD7711A also
offers a software programmable standby mode that reduces idle
power consumption to typically 7 mW.
THEORY OF OPERATIONThe general block diagram of a sigma-delta ADC is shown in
Figure 4. It contains the following elements:A sample-hold amplifier.A differential amplifier or subtracter.An analog low-pass filter.A 1-bit A/D converter (comparator).A 1-bit DAC.A digital low-pass filter.
Figure 4.General Sigma-Delta ADC
In operation, the analog signal sample is fed to the subtracter,
along with the output of the 1-bit DAC. The filtered difference
signal is fed to the comparator, whose output samples the differ-
ence signal at a frequency many times that of the analog signal
sampling frequency (oversampling).
Oversampling is fundamental to the operation of sigma-delta
ADCs. Using the quantization noise formula for an ADC:
SNR = (6.02 · number of bits + 1.76) dB,
The AD7711A samples the input signal at a frequency of
19.5kHz or greater (see Table III). As a result, the quantization
noise is spread over a much wider frequency than that of the
band of interest. The noise in the band of interest is reduced
still further by analog filtering in the modulator loop, which
shapes the quantization noise spectrum to move most of the
noise energy to frequencies outside the bandwidth of interest.
The noise performance is thus improved from this 1-bit level to
the performance outlined in Tables I and II and in Figure 2.
The output of the comparator provides the digital input for the
1-bit DAC, so that the system functions as a negative feedback
loop that tries to minimize the difference signal. The digital
data that represents the analog input voltage is contained in the
duty cycle of the pulse train appearing at the output of the
comparator. It can be retrieved as a parallel binary data word
using a digital filter.
Sigma-delta ADCs are generally described by the order of the
analog low-pass filter. A simple example of a first order sigma-
delta ADC is shown in Figure 5. This contains only a first
order low-pass filter or integrator. It also illustrates the deriva-
tion of the alternative name for these devices: Charge Balancing
ADCs.
Figure 5.Basic Charge-Balancing ADC
It consists of a differential amplifier (whose output is the differ-
ence between the analog input and the output of a 1-bit DAC),
an integrator and a comparator. The term, charge balancing,
comes from the fact that this system is a negative feedback loop
that tries to keep the net charge on the integrator capacitor at
zero by balancing charge injected by the input voltage with
charge injected by the 1-bit DAC. When the analog input is
zero, the only contribution to the integrator output comes from
the 1-bit DAC. For the net charge on the integrator capacitor
to be zero, the DAC output must spend half its time at +FS
and half its time at –FS. Assuming ideal components, the duty
cycle of the comparator will be 50%.
When a positive analog input is applied, the output of the 1-bit
DAC must spend a larger proportion of the time at +FS, so the
duty cycle of the comparator increases. When a negative input
voltage is applied, the duty cycle decreases.
The AD7711A uses a second order sigma-delta modulator and
a digital filter that provides a rolling average of the sampled
output. After power-up, or if there is a step change in the input
voltage, there is a settling time that must elapse before valid
data is obtained.
AD7711A
Input Sample RateThe modulator sample frequency for the device remains at
fCLK IN/512 (19.5 kHz @ fCLK IN = 10 MHz) regardless of the
selected gain. However, gains greater than ·1 are achieved by a
combination of multiple input samples per modulator cycle and
a scaling of the ratio of reference capacitor to input capacitor.
As a result of the multiple sampling, the input sample rate of
the device varies with the selected gain (see Table III). The
effective input impedance is 1/C · fS where C is the input sam-
pling capacitance and fS is the input sample rate.
Table III.Input Sampling Frequency vs. Gain1fCLK IN/256 (39 kHz @ fCLK IN = 10 MHz)
22 ·
44 ·
DIGITAL FILTERINGThe AD7711A’s digital filter behaves like a similar analog filter,
with a few minor differences.
First, since digital filtering occurs after the A-to-D conversion
process, it can remove noise injected during the conversion
process. Analog filtering cannot do this.
On the other hand, analog filtering can remove noise superim-
posed on the analog signal before it reaches the ADC. Digital
filtering cannot do this and noise peaks riding on signals near
full scale have the potential to saturate the analog modulator
and digital filter, even though the average value of the signal is
within limits. To alleviate this problem, the AD7711A has
overrange headroom built into the sigma-delta modulator and
digital filter which allows overrange excursions of 5% above the
analog input range. If noise signals are larger than this, consid-
eration should be given to analog input filtering, or to reducing
the input channel voltage so that its full scale is half that of the
analog input channel full scale. This will provide an overrange
capability greater than 100% at the expense of reducing the
dynamic range by 1 bit (50%).
Filter CharacteristicsThe cutoff frequency of the digital filter is determined by the
value loaded to bits FS0 to FS11 in the control register. At the
maximum clock frequency of 10 MHz, the minimum cutoff
frequency of the filter is 2.58 Hz while the maximum program-
mable cutoff frequency is 269 Hz.
Figure 6 shows the filter frequency response for a cutoff fre-
quency of 2.62 Hz, which corresponds to a first filter notch fre-
quency of 10 Hz. This is a (sinx/x)3 response (also called sinc3)
that provides >100 dB of 50 Hz and 60 Hz rejection. Program-
ming a different cutoff frequency via FS0–FS11 does not alter
the profile of the filter response, it changes the frequency of the
notches as outlined in the Control Register section.
Figure 6.Frequency Response of AD7711A Filter
Since the AD7711A contains this on-chip, low-pass filtering,
there is a settling time associated with step function inputs, and
data on the output will be invalid after a step change until the
settling time has elapsed. The settling time depends upon the
notch frequency chosen for the filter. The output data rate
equates to this filter notch frequency, and the settling time of
the filter to a full-scale step input is four times the output data
period. In applications using both input channels, the settling
time of the filter must be allowed to elapse before data from the
second channel is accessed.
Post FilteringThe on-chip modulator provides samples at a 19.5 kHz output
rate. The on-chip digital filter decimates these samples to
provide data at an output rate that corresponds to the pro-
grammed first notch frequency of the filter. Since the output
data rate exceeds the Nyquist criterion, the output rate for a
given bandwidth will satisfy most application requirements.
However, there may be some applications which require a
higher data rate for a given bandwidth and noise performance.
Applications that need this higher data rate will require some
post filtering following the digital filter of the AD7711A.
For example, if the required bandwidth is 7.86 Hz but the re-
quired update rate is 100 Hz, the data can be taken from the
AD7711A at the 100 Hz rate giving a –3 dB bandwidth of
26.2 Hz. Post filtering can be applied to this to reduce the band-
width and output noise, to the 7.86 Hz bandwidth level, while
maintaining an output rate of 100 Hz.
Post filtering can also be used to reduce the output noise from
the device for bandwidths below 2.62 Hz. At a gain of 128, the
output rms noise is 250 nV. This is essentially device noise or
white noise, and since the input is chopped, the noise has a flat
frequency response. By reducing the bandwidth below 2.62 Hz,
the noise in the resultant passband can be reduced. A reduction
in bandwidth by a factor of two results in a √2 reduction in the
output rms noise. This additional filtering will result in a longer
settling time.