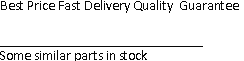
AD590JH ,2-Wire/ Current Output Temperature TransducerSPECIFICATIONS S Model AD590J AD590KMin Typ Max Min Typ Max UnitsABSOLUTE MAXIMUM RATINGSForward Vo ..
AD590KF ,Two-Terminal IC Temperature TransducerFEATURESLinear Current Output: 1 mA/KWide Range: –558C to +1508CProbe Compatible Ceramic Sensor Pac ..
AD590KH ,Two-Terminal IC Temperature Transducercharacteristics also make theAD590 easy to multiplex: the current can be switched by aCMOS multiple ..
AD590LF ,Two-Terminal IC Temperature TransducerTwo-Terminal ICaTemperature TransducerAD590PIN DESIGNATIONS
AD590LH ,Two-Terminal IC Temperature TransducerSpecifications subject to change without notice.
AD590LH ,Two-Terminal IC Temperature Transducerapplications.The device is insensitive to voltage drops over long lines due toits high impedance cu ..
AD9849AKSTRL ,12-Bit, 30 MHz CCD Signal Processor with Integrated Timing DriverSPECIFICATIONS DVDD2 = 5.25 V (AD9849), C = 20 pF, unless otherwise noted.)L Parameter Symbol Min T ..
AD9849AKSTZ ,12-Bit, 30 MHz CCD Signal Processor with Integrated Timing DriverAPPLICATIONS–20°C to +85°C.Digital Still CamerasFUNCTIONAL BLOCK DIAGRAMVRT VRBVREF46dB 2dB TO 36d ..
AD9849KST ,CCD Signal Processors with Integrated Timing DriverSPECIFICATIONSParameter Min Typ Max UnitTEMPERATURE RANGEOperating –20 +85 °CStorage –65 +150 °CMAX ..
AD9849KST ,CCD Signal Processors with Integrated Timing DriverSPECIFICATIONS DVDD2 = 5.25 V [AD9849], C = 20 pF, unless otherwise noted.)L Parameter Symbol Min T ..
AD9850BRS ,CMOS, 125 MHz Complete DDS SynthesizerCHARACTERISTICSClock Output Duty Cycle (Clk Gen. Config.) +25°C IV 50 ± 10 %–2–REV. EAD9850AD9850BR ..
AD9850BRS. ,CMOS, 125 MHz Complete DDS SynthesizerGENERAL DESCRIPTION combination thereof. The AD9850 also contains a high speedThe AD9850 is a highl ..
AD590JH-AD590KF-AD590KH-AD590LF-AD590LH-AD590MF-AD590MH
Two-Terminal IC Temperature Transducer
PIN DESIGNATIONSREV.B
Two-Terminal ICemperature Transducer
FEATURES
Linear Current Output: 1 mA/K
Wide Range: –558C to +1508C
Probe Compatible Ceramic Sensor Package
Two Terminal Device: Voltage In/Current Out
LaserTrimmedto60.58C
CalibrationAccuracy(AD590M)
Excellent Linearity: 60.38C Over Full Range (AD590M)
Wide Power Supply Range: +4 V to +30 V
Sensor Isolation from Case
Low Cost
PRODUCT DESCRIPTIONThe AD590 is a two-terminal integrated circuit temperature
transducer that produces an output current proportional to
absolute temperature. For supply voltages between +4 V and
+30 V the device acts as a high impedance, constant current
regulator passing 1 μA/K. Laser trimming of the chip’s thin-film
resistors is used to calibrate the device to 298.2 μA output at
298.2K (+25°C).
The AD590 should be used in any temperature sensing applica-
tion below +150°C in which conventional electrical temperature
sensors are currently employed. The inherent low cost of a
monolithic integrated circuit combined with the elimination of
support circuitry makes the AD590 an attractive alternative for
many temperature measurement situations. Linearization
circuitry, precision voltage amplifiers, resistance measuring
circuitry and cold junction compensation are not needed in
applying the AD590.
In addition to temperature measurement, applications include
temperature compensation or correction of discrete compo-
nents, biasing proportional to absolute temperature, flow
rate measurement, level detection of fluids and anemometry.
The AD590 is available in chip form making, it suitable for
hybrid circuits and fast temperature measurements in protected
environments.
The AD590 is particularly useful in remote sensing applications.
The device is insensitive to voltage drops over long lines due to
its high impedance current output. Any well insulated twisted
pair is sufficient for operation hundreds of feet from the
receiving circuitry. The output characteristics also make the
AD590 easy to multiplex: the current can be switched by a
CMOS multiplexer or the supply voltage can be switched by a
logic gate output.
PRODUCT HIGHLIGHTSThe AD590 is a calibrated two terminal temperature sensor
requiring only a dc voltage supply (+4 V to +30 V). Costly
transmitters, filters, lead wire compensation and linearization
circuits are all unnecessary in applying the device.State-of-the-art laser trimming at the wafer level in conjunc-
tion with extensive final testing ensures that AD590 units are
easily interchangeable.Superior interface rejection results from the output being a
current rather than a voltage. In addition, power require-
ments are low (1.5 mWs @ 5 V @ +25°C.) These features
make the AD590 easy to apply as a remote sensor.The high output impedance (>10 MΩ) provides excellent
rejection of supply voltage drift and ripple. For instance,
changing the power supply from 5 V to 10 V results in only
a 1 μA maximum current change, or 1°C equivalent error.The AD590 is electrically durable: it will withstand a forward
voltage up to 44 V and a reverse voltage of 20 V. Hence, sup-
plyirregularitiesorpinreversalwillnotdamagethedevice.
AD590–SPECIFICATIONSPOWER SUPPLY
OUTPUT
PACKAGE OPTIONS
NOTESThe AD590 has been used at –100°C and +200°C for short periods of measurement with no physical damage to the device. However, the absolute errors
specified apply to only the rated performance temperature range.Maximum deviation between +25°C readings after temperature cycling between –55°C and +150°C; guaranteed not tested.Conditions: constant +5 V, constant +125°C; guaranteed, not tested.Leakage current doubles every 10°C.
Specifications subject to change without notice.
Specifications shown in boldface are tested on all production units at final electrical test. Results from those tests are used to calculate outgoing quality levels.
All min and max specifications are guaranteed, although only those shown in boldface are tested on all production units.
(@ +258C and VS = +5 V unless otherwise noted)
AD590POWER SUPPLY
OUTPUT
PACKAGE OPTIONS
TEMPERATURE SCALE CONVERSION EQUATIONS =5(°F±32)K=°C+273.15=9°C+32°R=°F+459.7
AD590The 590H has 60 μ inches of gold plating on its Kovar leads and
Kovar header. A resistance welder is used to seal the nickel cap
to the header. The AD590 chip is eutectically mounted to the
header and ultrasonically bonded to with 1 MIL aluminum
wire. Kovar composition: 53% iron nominal; 29% ±1% nickel;
17% ± 1% cobalt; 0.65% manganese max; 0.20% silicon max;
0.10%aluminummax;0.10%magnesium max; 0.10% zirco-
nium max; 0.10% titaniummax;0.06%carbonmax.
The 590F is a ceramic package with gold plating on its Kovar
leads, Kovar lid, and chip cavity. Solder of 80/20 Au/Sn com-
position is used for the 1.5 mil thick solder ring under the lid.
The chip cavity has a nickel underlay between the metalization
and the gold plating. The AD590 chip is eutectically mounted
in the chip cavity at 410°C and ultrasonically bonded to with 1
mil aluminum wire. Note that the chip is in direct contact with
the ceramic base, not the metal lid. When using the AD590 in
die form, the chip substrate must be kept electrically isolated,
(floating), for correct circuit operation.
METALIZATION DIAGRAM
CIRCUIT DESCRIPTION1The AD590 uses a fundamental property of the silicon transis-
tors from which it is made to realize its temperature propor-
tional characteristic: if two identical transistors are operated at a
constant ratio of collector current densities, r, then the differ-
ence in their base-emitter voltage will be (kT/q)(In r). Since
both k, Boltzman’s constant and q, the charge of an electron,
are constant, the resulting voltage is directly proportional to
absolute temperature (PTAT).
In the AD590, this PTAT voltage is converted to a PTAT cur-
rent by low temperature coefficient thin-film resistors. The total
current of the device is then forced to be a multiple of this
PTAT current. Referring to Figure 1, the schematic diagram of
the AD590, Q8 and Q11 are the transistors that produce the
PTAT voltage. R5 and R6 convert the voltage to current. Q10,
whose collector current tracks the colletor currents in Q9 and
Q11, supplies all the bias and substrate leakage current for the
rest of the circuit, forcing the total current to be PTAT. R5 and
R6 are laser trimmed on the wafer to calibrate the device at
+25°C.
Figure 2 shows the typical V–I characteristic of the circuit at
+25°C and the temperature extremes.
Figure 1.Schematic Diagram
Figure 2.V–I Plot
EXPLANATION OF TEMPERATURE SENSOR
SPECIFICATIONSThe way in which the AD590 is specified makes it easy to apply
in a wide variety of different applications. It is important to
understand the meaning of the various specifications and the
effects of supply voltage and thermal environment on accuracy.
The AD590 is basically a PTAT (proportional to absolute
temperature)1 current regulator. That is, the output current is
equal to a scale factor times the temperature of the sensor in
degrees Kelvin. This scale factor is trimmed to 1 μA/K at the
factory, by adjusting the indicated temperature (i.e., the output
current) to agree with the actual temperature. This is done with
5 V across the device at a temperature within a few degrees of
+25°C (298.2K). The device is then packaged and tested for
accuracy over temperature.
CALIBRATION ERRORAt final factory test the difference between the indicated
temperature and the actual temperature is called the calibration
error. Since this is a scale factory error, its contribution to the
total error of the device is PTAT. For example, the effect of the
1°C specified maximum error of the AD590L varies from 0.73°C
at –55°C to 1.42°C at 150°C. Figure 3 shows how an exagger-
ated calibration error would vary from the ideal over temperature.
Figure 3.Calibration Error vs. Temperature
The calibration error is a primary contributor to maximum total
error in all AD590 grades. However, since it is a scale factor
error, it is particularly easy to trim. Figure 4 shows the most
elementary way of accomplishing this. To trim this circuit the
temperature of the AD590 is measured by a reference tempera-
ture sensor and R is trimmed so that VT = 1 mV/K at that
temperature. Note that when this error is trimmed out at one
temperature, its effect is zero over the entire temperature range.
In most applications there is a current-to-voltage conversion
resistor (or, as with a current input ADC, a reference) that can
be trimmed for scale factor adjustment.
ERROR VERUS TEMPERATURE: WITH CALIBRATION
ERROR TRIMMED OUTEach AD590 is tested for error over the temperature range with
the calibration error trimmed out. This specification could also
be called the “variance from PTAT” since it is the maximum
difference between the actual current over temperature and a
PTAT multiplication of the actual current at 25°C. This error
consists of a slope error and some curvature, mostly at the
temperature extremes. Figure 5 shows a typical AD590K
temperature curve before and after calibration error trimming.
Figure 5.Effect to Scale Factor Trim on Accuracy
ERROR VERSUS TEMPERATURE: NO USER TRIMSUsing the AD590 by simply measuring the current, the total
error is the “variance from PTAT” described above plus the
effect of the calibration error over temperature. For example the
AD590L maximum total error varies from 2.33°C at –55°C to
3.02°C at 150°C. For simplicity, only the large figure is shown
on the specification page.
NONLINEARITYNonlinearity as it applies to the AD590 is the maximum
deviation of current over temperature from a best-fit straight
line. The nonlinearity of the AD590 over the –55°C to +150°C
range is superior to all conventional electrical temperature
sensors such as thermocouples. RTDs and thermistors. Figure 6
shows the nonlinearity of the typical AD590K from Figure 5.
Figure 6.Nonlinearity
Figure 7A shows a circuit in which the nonlinearity is the major
contributor to error over temperature. The circuit is trimmed by
adjusting R1 for a 0 V output with the AD590 at 0°C. R2 is then
adjusted for 10 V out with the sensor at 100°C. Other pairs of
temperatures may be used with this procedure as long as they
AD590Figure 7A.Two Temperature Trim
Figure 7B.Typical Two-Trim Accuracy
VOLTAGE AND THERMAL ENVIRONMENT EFFECTSThe power supply rejection specifications show the maximum
expected change in output current versus input voltage changes.
The insensitivity of the output to input voltage allows the use of
unregulated supplies. It also means that hundreds of ohms of
resistance (such as a CMOS multiplexer) can be tolerated in
series with the device.
It is important to note that using a supply voltage other than 5 V
does not change the PTAT nature of the AD590. In other
words, this change is equivalent to a calibration error and can be
removed by the scale factor trim (see previous page).
TheAD590specificationsareguaranteedforuseinalowthermal
resistance environment with 5 V across the sensor. Large
changes in the thermal resistance of the sensor’s environment
will change the amount of self-heating and result in changes in
the output which are predictable but not necessarily desirable.
The thermal environment in which the AD590 is used deter-
mines two important characteristics: the effect of self heating
and the response of the sensor with time.
Figure 8.Thermal Circuit Model
Figure 8 is a model of the AD590 which demonstrates these
characteristics. As an example, for the TO-52 package, θJC is
the thermal resistance between the chip and the case, about
26°C/watt. θCA is the thermal resistance between the case and
thermal connection. Power source P represents the power
dissipated on the chip. The rise of the junction temperature, TJ,
above the ambient temperature TA is: TJ−TA=P(θJC+θCA) Equation 1
Table I gives the sum of θJC and θCA for several common
thermal media for both the “H” and “F” packages. The heatsink
used was a common clip-on. Using Equation 1, the temperature
rise of an AD590 “H” package in a stirred bath at +25°C, when
driven with a 5 V supply, will be 0.06°C. However, for the same
conditions in still air the temperature rise is 0.72°C. For a given
supply voltage, the temperature rise varies with the current and
is PTAT. Therefore, if an application circuit is trimmed with
the sensor in the same thermal environment in which it will be
used, the scale factor trim compensates for this effect over the
entire temperature range.
Table I.Thermal ResistancesNote: τ is dependent upon velocity of oil; average of several velocities listed
above.Air velocity ≅ 9 ft./sec.The time constant is defined as the time required to reach 63.2% of an
instantaneous temperature change.
The time response of the AD590 to a step change in tempera-
ture is determined by the thermal resistances and the thermal
capacities of the chip, CCH, and the case, CC. CCH is about
0.04 watt-sec/°C for the AD590. CC varies with the measured
medium since it includes anything that is in direct thermal
contact with the case. In most cases, the single time constant
exponential curve of Figure 9 is sufficient to describe the time
response, T (t). Table I shows the effective time constant, τ, for
several media.